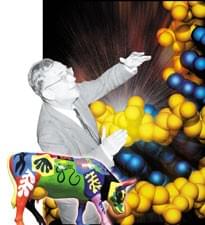
Circa 2000
A 1940 paper by Gamow and Mario Schoenberg was the first in a subject we now call particle astrophysics. The two authors presciently speculated that neutrinos could play a role in the cooling of massive collapsing stars. They named the neutrino reaction the Urca process, after a well known Rio de Janeiro casino. This name might seem a strange choice, but not to Gamow, a legendary prankster who once submitted a paper to Nature in which he suggested that the Coriolis force might account for his observation that cows chewed clockwise in the Northern Hemisphere and counterclockwise in the Southern Hemisphere.
In the 1940s Gamow began to attack, with his colleague Ralph Alpher, the problem of the origin of the chemical elements. Their first paper on the subject appeared in a 1948 issue of the Physical Review. At the last minute Gamow, liking the sound of ‘alpha, beta, gamma’, added his old friend Hans Bethe as middle author in absentia (Bethe went along with the joke, but the editors did not). Gamow and Alpher, with Robert Herman, then pursued the idea of an extremely hot neutron-dominated environment. They envisioned the neutrons decaying into protons, electrons and anti-neutrinos and, when the universe had cooled sufficiently, the neutrons and protons assembling heavier nuclei. They even estimated the photon background that would be necessary to account for nuclear abundances, suggesting a residual five-degree background radiation.
We now realize that their scheme was incorrect. The Universe began with roughly equal numbers of protons and neutrons. Collisions with electrons, positrons, neutrinos and anti-neutrinos are more important than neutron decay, and the absence of stable nuclei with atomic numbers of five and eight creates a barrier to further fabrication in the early Universe. Nevertheless Alpher, Gamow and Herman’s work was the first serious attempt to discuss the observable consequences of a big bang and the basic framework was correct. Ironically, the term ‘Big Bang’ was coined by Fred Hoyle, an advocate of a steady-state model of the universe, to make fun of Gamow’s efforts.