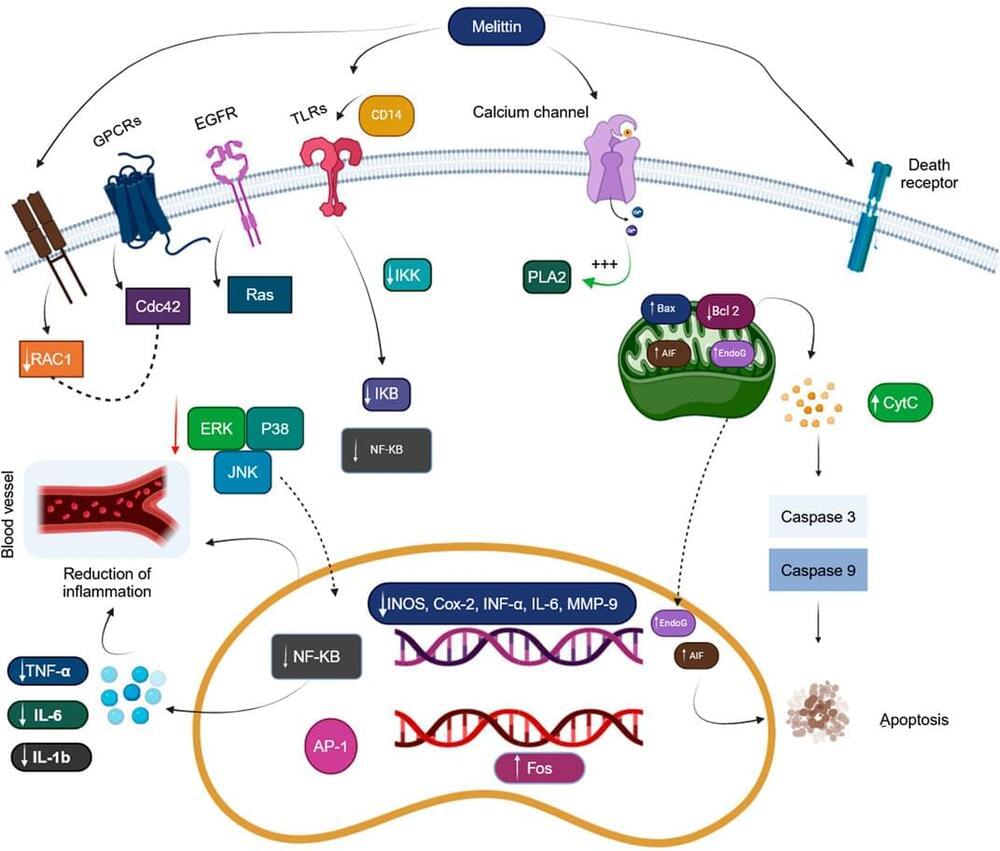
After the bee stings a person, pain, and swelling occur in this place, due to the effects of bee venom (BV). This is not a poison in the total sense of the word because it has many benefits, and this is due to its composition being rich in proteins, peptides, enzymes, and other types of molecules in low concentrations that show promise in the treatment of numerous diseases and conditions. BV has also demonstrated positive effects against various cancers, antimicrobial activity, and wound healing versus the human immunodeficiency virus (HIV). Even though topical BV therapy is used to varying degrees among countries, localized swelling or itching are common side effects that may occur in some patients. This review provides an in-depth analysis of the complex chemical composition of BV, highlighting the diverse range of bioactive compounds and their therapeutic applications, which extend beyond the well-known anti-inflammatory and pain-relieving effects, showcasing the versatility of BV in modern medicine. A specific search strategy was followed across various databases; Web of sciences, Scopus, Medline, and Google Scholar including in vitro and in vivo clinical studies.to outline an overview of BV composition, methods to use, preparation requirements, and Individual consumption contraindications. Furthermore, this review addresses safety concerns and emerging approaches, such as the use of nanoparticles, to mitigate adverse effects, demonstrating a balanced and holistic perspective. Importantly, the review also incorporates historical context and traditional uses, as well as a unique focus on veterinary applications, setting it apart from previous works and providing a valuable resource for researchers and practitioners in the field.
Bees are commercially beneficial insects that have been around since the Cretaceous age of the Mesozoic Era. They also help fertilize many different crops. Bees are helpful, but their capacity to administer excruciating and poisonous stings constitutes a risk. Thankfully, most honeybees are not hostile to people and only resort to violence if they perceive danger (Pucca et al., 2019). Apis mellifera is the most often used honeybee species for agricultural pollination globally. All bee products, particularly venom, and honey, have been used for centuries, and their medicinal properties have been described in holy writings such as the Bible and the Quran (Ali, 2024; Dinu et al., 2024). Bee venom (BV) treatment involves injecting honeybee venom into the human body to cure various ailments. For over 5,000 years, this technique has been used in complementary therapies.