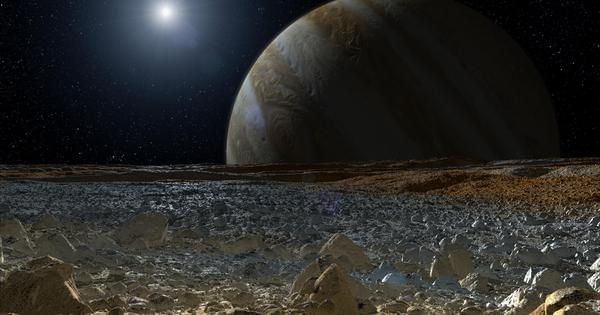
A scientist who loves to write, can do it well, and can share the excitement of the scientific pursuit is incredibly rare. Kevin Peter Hand 0, Deputy Project Scientist, Europa and Director of the JPL Ocean Worlds Lab is that rare person who can do all these things. In his incredible book Alien Oceans: The Search for Life in the Depths of Space 0, he explains that “We know that the laws of physics, the principles of chemistry, and the principles of geology all work beyond Earth. We’ve explored other worlds and observed that these sciences are universal. When it comes to biology, however, we have yet to make that leap.”
If you want to learn about how the intersection of numerous areas of science are helping inform our understanding of the oceans, space, and ourselves, Alien Oceans is by far one of the most clearly written books on the topic. As Kevin notes, he wrote the book he wishes he could have read in college. Kevin will teach you and inspire you and explain complicated scientific topics in ways nearly anyone can understand. Not only is it a book about his areas of expertise, it is also a wonderful window into the way scientists and engineers think about solving real world problems and applying basic knowledge. For example, Kevin notes in this interview that “Making measurements is where the creativity of science meets the hard reality of engineering.” I read a lot of books on science written for a broad audience, and this book, by far is among the very best I have ever read. More than anything else what came through in Kevin’s writing is excitement about finding out what is true.
What inspired you to write this book?