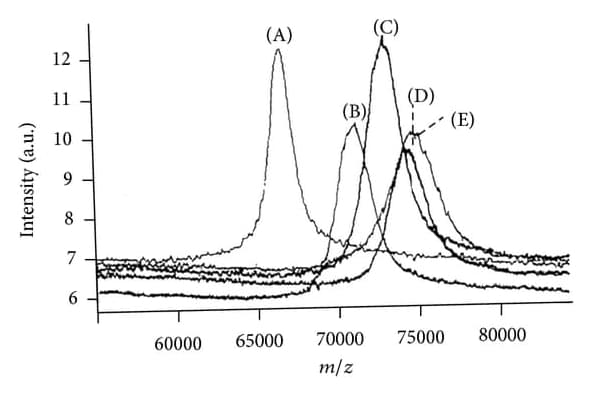
Glucose monitoring with mass spectrometry circa 2013.
Diabetes is a common endocrine disorder characterized by hyperglycemia leading to nonenzymatic glycation of proteins, responsible for chronic complications. The development of mass spectrometric techniques able to give highly specific and reliable results in proteome field is of wide interest for physicians, giving them new tools to monitor the disease progression and the possible complications related to diabetes, as well as the effectiveness of therapeutic treatments. This paper reports and discusses some of the data pertaining protein glycation in diabetic subjects obtained by matrix-assisted laser desorption ionization (MALDI) mass spectrometry (MS). The preliminary studies carried out by in vitro protein glycation experiments show clear differences in molecular weight of glycated and unglycated proteins. Then, the attention was focused on plasma proteins human serum albumin (HSA) and immunoglobulin G (IgG). Enzymatic degradation products of in vitro glycated HSA were studied in order to simulate the in vivo enzymatic digestion of glycated species by the immunological system leading to the highly reactive advanced glycation end-products (AGEs) peptides. Further studies led to the evaluation of glycated Apo A-I and glycated haemoglobin levels. A different MALDI approach was employed for the identification of markers of disease in urine samples of healthy, diabetic, nephropathic, and diabetic-nephropathic subjects.
Diabetes is usually considered as a disease related to glucose dysmetabolism. In particular, type 1 diabetes is a chronic disease related to metabolism of carbohydrates, fats, and proteins, caused by the lack of insulin. It results from the marked and progressive inability of the pancreas to secrete insulin, due to autoimmune destruction of the beta cells. On the other hand, type 2 diabetes is caused by islet beta cells being unable to secrete adequate insulin in response to varying degrees of overnutrition, inactivity, obesity, and insulin resistance. Nowadays, the burden of diabetes is enormous, due to its increasing global prevalence and the occurrence of chronic complications affecting many tissues (retinopathy, nephropathy, neuropathy, and cardiovascular disease) reflecting in high direct and indirect costs [1].
This view may be seen somehow reductive, considering that the side effects of the previous mechanisms are at systemic level, and, taking into account the high complexity of the biological environment, it necessarily reflects on a high number of different pathological pathways, catalyzed by the glucose dysmetabolism. In this context, considering the Maillard reaction pattern [2], proteins seem to be at first sight the target of the glucose molecules circulating at high level in diabetes, and only some papers gave contradictory results about the reactivity of sugar with respect to DNA [3, 4].