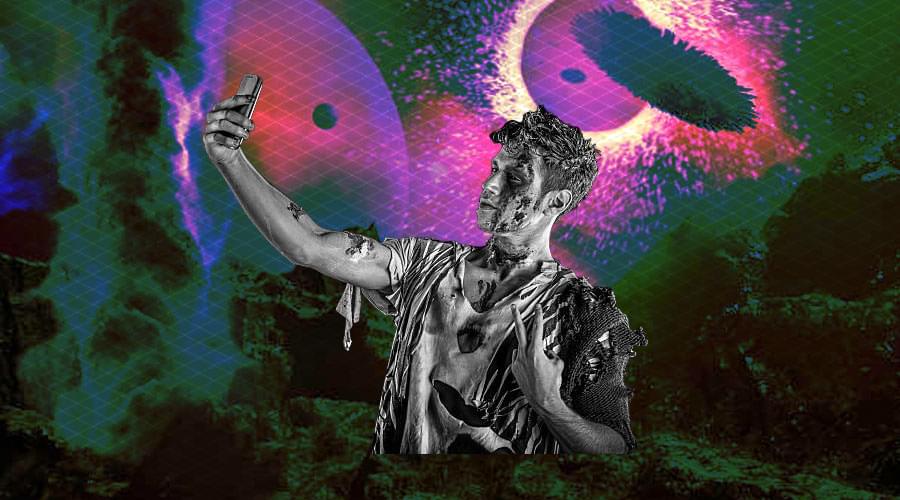
So, Artificial intelligence predicts selfies would dominate, ghoulish humans, holding mobiles, at the end of the earth, an event that would destroy every sign of life. Indeed, it is hypothetical and difficult to imagine the situation. An AI image generator, Midjourney, an obscure but close associate of Open AI, imagined a few of them revealing how scary they can be. Shared by a tik-tok account, @Robot Overloads, the images were hellish in tone and gory in substance. The images generated depict disfigured human beings with eyes as big as rat holes and fingers long enough to scoop out curdled blood from creatures of another world. These frames artificial intelligence has generated go beyond the portrayal of annihilation. Firstly, they are cut off from reality, and secondly, they are very few. The end of the world is billion years away when selfies would become a fossilized concept and humans are considered biological ancestors of cyborgs.
The pictures are stunning though in the sense that the elements like huge explosions going off in the background while a man maniacally staring into the camera are included in one frame. The imaginative spark of artificial intelligence should really be appreciated here. Perhaps it must have taken a hint or two from images of people taking selfies in the backdrop of accidents and natural calamities, to use them as click baits. Apparently, image generators give the users the power to visualize their imagination, how much ever removed from reality. However, the netizens are finding them captivating pleasantly, so much so that one of them wonders if they are from nibiru or planet X theories!! That one tik-tok video has got more than 12.7 million views and the reply, “OK no more sleeping,” posted by a Tik Tok user summarises, more than anything, the superficiality of melodramatic AI’s image generating capability.