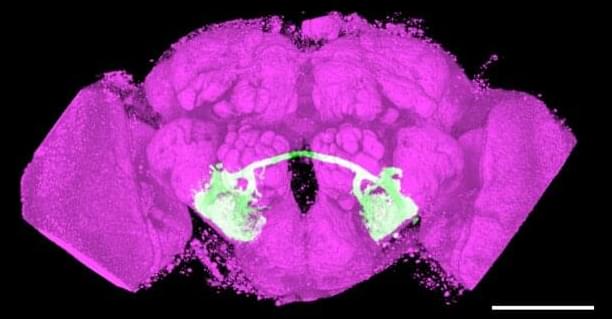
When it comes to courtship, it is important to ensure that one is interacting with a member of the same species. Animals use multiple sensory systems to confirm that potential mates are indeed suitable, with acoustic communication playing an important role in their decision making.
Although these differences have previously been reported at the behavioral level, it is not known how the neuronal circuitry underlying this decision-making has diverged between species. Now, in a new publication in Scientific Reports, a research group at Nagoya University in Japan has investigated how the auditory processing pathway has evolved and diverged between fruit fly species.
Males of several species of Drosophila (fruit flies), which are regularly used in neuroscience research, vibrate their wings rhythmically during courtship, producing a courtship song. The temporal components of these songs differ between species, allowing female flies to distinguish between potential mates.