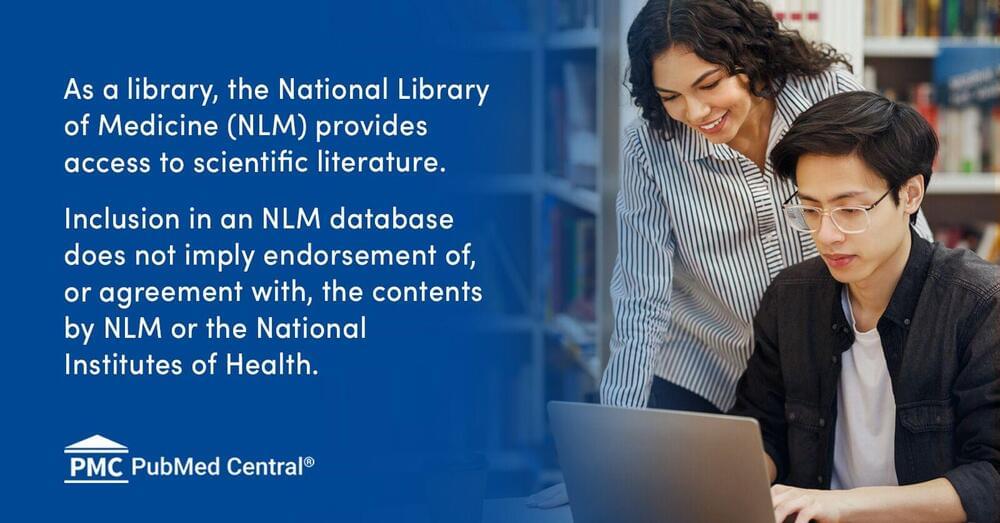
Patients suffering from diseased and injured organs are often treated with transplanted organs, and this treatment has been in use for over 50 years. In 1955, the kidney became the first entire organ to be replaced in a human, when Murray transplanted this organ between identical twins. Several years later, Murray performed an allogeneic kidney transplant from a non-genetically identical patient into another. This transplant, which overcame the immunologic barrier, marked a new era in medicine and opened the door for use of transplantation as a means of therapy for different organ systems.
As modern medicine increases the human lifespan, the aging population grows, and the need for donor organs grows with it, because aging organs are generally more prone to failure. However, there is now a critical shortage of donor organs, and many patients in need of organs will die while waiting for transplants. In addition, even if an organ becomes available, rejection of organs is still a major problem in transplant patients despite improvements in the methods used for immunosuppression following the transplant procedure. Even if rejection does not occur, the need for lifelong use of immunosuppressive medications leads to a number of complications in these patients.
These problems have led physicians and scientists to look to new fields for alternatives to organ transplantation. In the 1960s, a natural evolution occurred in which researchers began to combine new devices and materials sciences with cell biology, and a new field that is now termed tissue engineering was born. As more scientists from different fields came together with the common goal of tissue replacement, the field of tissue engineering became more formally established. Tissue engineering is now defined as an interdisciplinary field which applies the principles of engineering and life sciences towards the development of biological substitutes that aim to maintain, restore or improve tissue function.