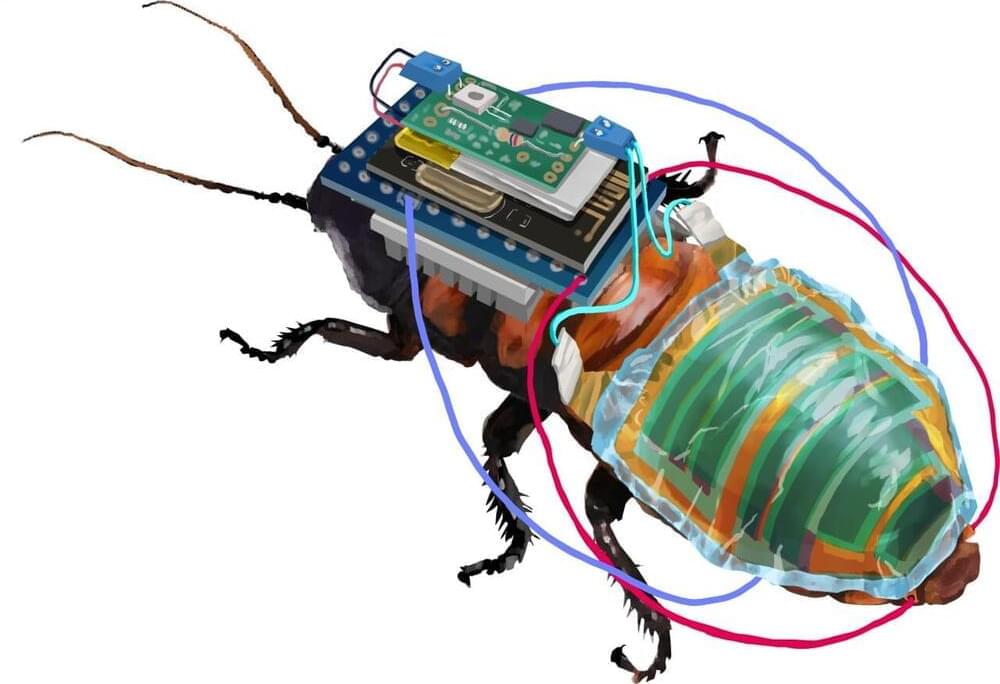
An international team led by researchers at the RIKEN Cluster for Pioneering Research (CPR) has engineered a system for creating remote controlled cyborg cockroaches, equipped with a tiny wireless control module that is powered by a rechargeable battery attached to a solar cell. Despite the mechanic devices, ultrathin electronics and flexible materials allow the insects to move freely. These achievements, reported in the scientific journal npj Flexible Electronics on September 5, will help make the use of cyborg insects a practical reality.
Researchers have been trying to design cyborg insects—part insect, part machine—to help inspect hazardous areas or monitor the environment. However, for the use of cyborg insects to be practical, handlers must be able to control them remotely for long periods of time. This requires wireless control of their leg segments, powered by a tiny rechargeable battery. Keeping the battery adequately charged is fundamental—nobody wants a suddenly out-of-control team of cyborg cockroaches roaming around. While it’s possible to build docking stations for recharging the battery, the need to return and recharge could disrupt time-sensitive missions. Therefore, the best solution is to include an on-board solar cell that can continuously ensure that the battery stays charged.
All of this is easier said than done. To successfully integrate these devices into a cockroach that has limited surface area required the research team to develop a special backpack, ultrathin organic solar cell modules, and an adhesion system that keeps the machinery attached for long periods of time while also allowing natural movements.