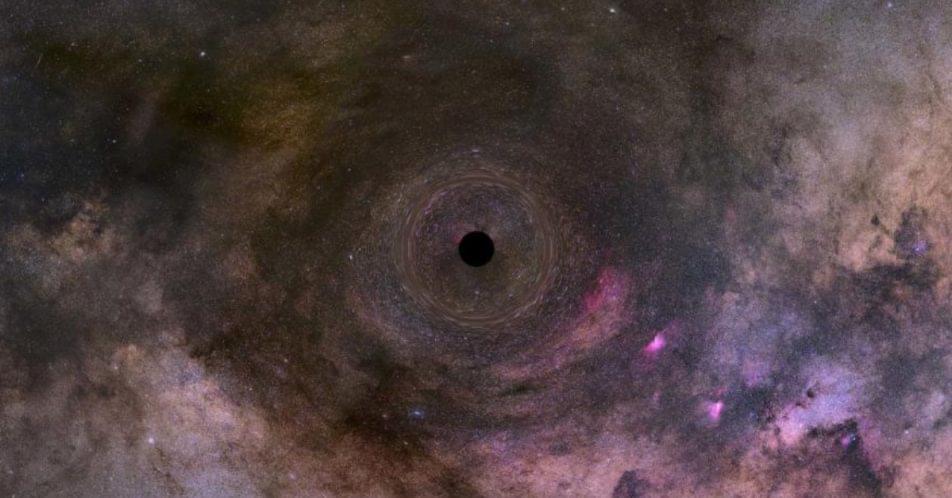
In late May, a collaborative study, led by Kailash Suhu, was published claiming that they had managed to identify the first ever isolated black hole, identified by shorthand as OB11046. While by itself, this discovery presents no new information with regards to their nature, it highlights the staggering progress we’ve made in recent years in detecting these bodies.
Previously, black hole detection was very much limited by the fact that they do not emit, nor reflect any detectable electromagnetic radiation. As such, astronomers were only able to infer their presence via two mechanisms.
The first is by tracking the orbits of nearby celestial bodies and observe whether their motion can be modelled by the forces experienced by their neighbours. Any unusual motion can usually be explained by a nearby black hole contributions. The second requires the black hole to form an accretion disk. As matter is caught in the intense gravitational field, it orbits the black hole and is accelerated to intense velocities, causing the material to emit certain wavelengths of high energy electromagnetic radiation, such as x-rays.