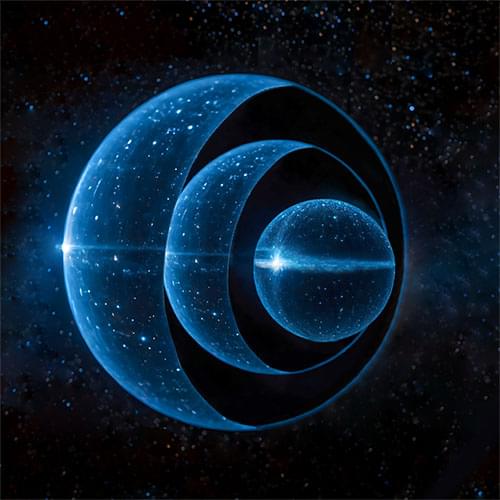
The interior of black holes remains a conundrum for science. In 1916, German physicist Karl Schwarzschild outlined a solution to Albert Einstein’s equations of general relativity, in which the center of a black hole consists of a so-called singularity, a point at which space and time no longer exist. Here, the theory goes, all physical laws, including Einstein’s general theory of relativity, no longer apply; the principle of causality is suspended.
This constitutes a great nuisance for science—after all, it means that no information can escape from a black hole beyond the so-called event horizon. This could be a reason why Schwarzschild’s solution did not attract much attention outside the theoretical realm—that is, until the first candidate for a black hole was discovered in 1971, followed by the discovery of the black hole in the center of our Milky Way in the 2000s, and finally the first image of a black hole, captured by the Event Horizon Telescope Collaboration in 2019.
In 2001, Pawel Mazur and Emil Mottola proposed a different solution to Einstein’s field equations that led to objects that they called gravitational condensate stars, or gravastars. Contrary to black holes, gravastars have several advantages from a theoretical astrophysics perspective.